Which PET biomarkers are most effective for tracking disease progression in HD?
Huntington’s Disease
Huntington’s disease (HD) is a rare, progressive neurodegenerative disorder inherited in an autosomal dominant manner. Symptoms typically emerge between the ages of 35 and 40, though in rare cases, juvenile-onset Huntington’s disease (JHD) can present before the age of 20 (Klöppel, 2009). The disease is characterized by a gradual decline in motor function, cognitive abilities, and neuropsychiatric health. The underlying cause of HD is a mutation in the HTT gene, where an expansion of CAG repeats results in the production of a toxic, mutant huntingtin protein (mHTT). When the CAG repeat count exceeds 39, the disease manifests, with higher repeat counts correlating with earlier onset and more rapid disease progression (Reiner, 2011; Wijeratne, 2021).
A key pathological feature of HD is the degeneration of medium spiny neurons (MSNs) in the striatum, accompanied by structural changes such as striatal atrophy. Neuroimaging, particularly magnetic resonance imaging (MRI) and positron emission tomography (PET), scans can detect early brain changes, often before the onset of motor symptoms, providing crucial insights into the progression of the disease and potential avenues for early intervention (Klöppel, 2009; Hobbs, 2024).
Although no disease-modifying therapies exist at present, ongoing research focuses on targeting mHTT aggregates, which play a central role in the disease’s pathology. PET imaging is instrumental in monitoring disease progression by visualizing various molecular and metabolic changes in the brain. These include alterations in glucose metabolism, dopaminergic activity, phosphodiesterase levels, and neuroinflammation (Cybulska, 2020). Additionally, the development of novel PET radioligands aimed at quantifying and tracking mHTT aggregates is underway, with the potential to become valuable tools in assessing the efficacy of new therapeutic approaches (Fazio, 2018; Cybulska, 2020; Dickmann, 2024).
Cerebral Glucose Metabolism
One of the earliest biomarkers of HD is striatal hypometabolism, which can be detected using [18F]fluorodeoxyglucose (FDG) PET imaging. This metabolic decline often occurs before clinical symptoms appear. Longitudinal studies have shown that glucose metabolism progressively decreases in both subcortical and cortical regions of the brain, including the striatum, thalamus, insula, posterior cingulate gyrus, prefrontal cortex, and occipital cortex (Fazio, 2018). Moreover, reductions in cortical glucose metabolism are linked to the worsening of cognitive deficits (Fazio, 2018).
In JHD, FDG uptake is lower in the striatum and entorhinal cortex compared to adult-onset HD. Conversely, individuals with adult-onset HD show more significant reductions in cortical areas, such as the left inferior parietal gyrus and left cingulate gyrus (Caligiuri, 2024). Longitudinal studies also reveal different patterns of metabolic decline, with adult-onset HD exhibiting a more consistent reduction in cortical FDG uptake, whereas JHD shows more variability (Caligiuri, 2024). Interestingly, PET studies have identified early thalamic hypermetabolism in premanifest HD gene carriers, which tends to decrease once clinical symptoms manifest (Feigin, 2007). This early thalamic hypermetabolism may reflect compensatory mechanisms, though its precise role remains unclear (Feigin, 2007; Hellem, 2021).
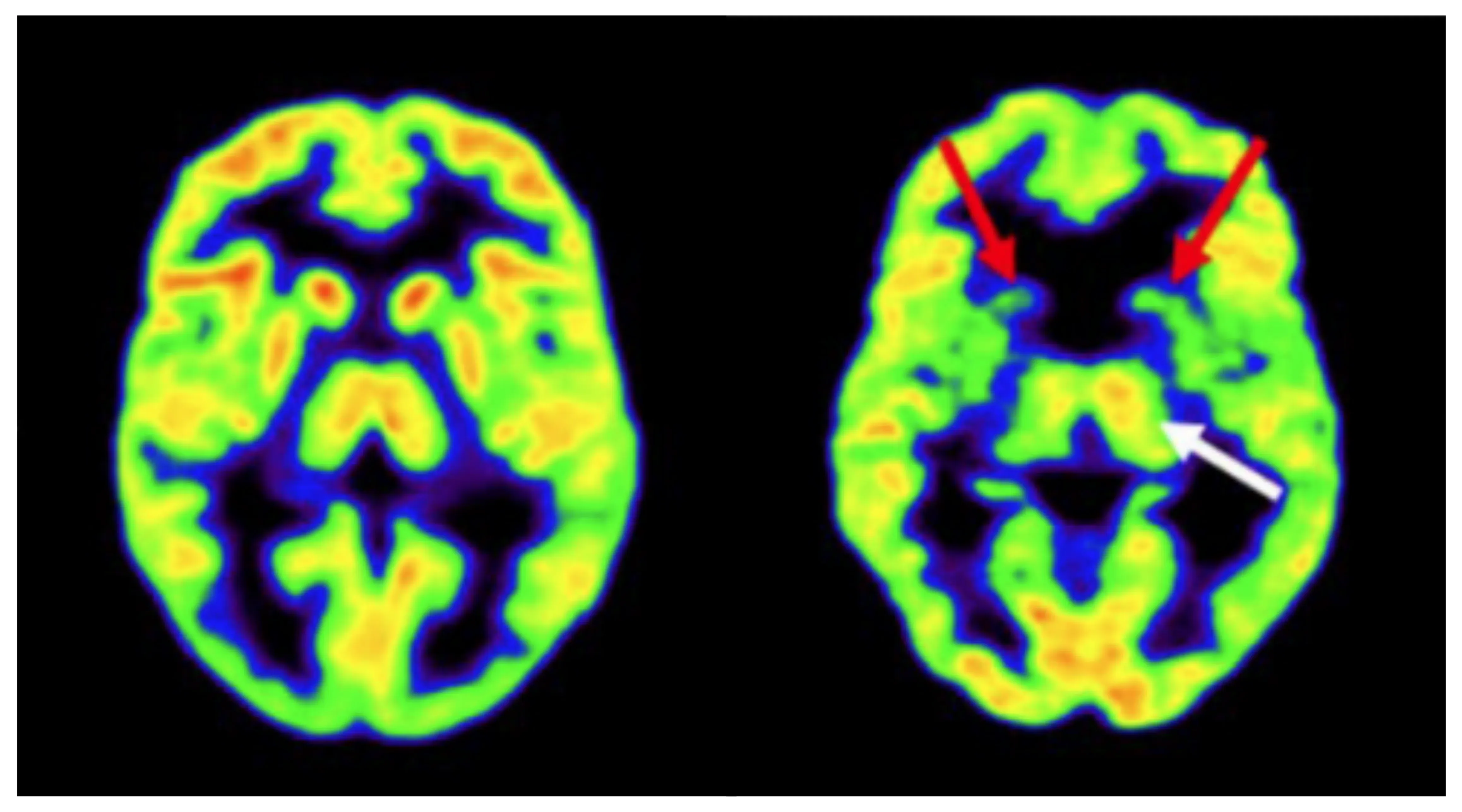
FDG PET scan of a healthy control (left) and a premanifest HD gene expansion carrier (right). The HD gene expansion carrier shows reduced bilateral striatal metabolism (red arrows) and a potential compensatory increase in metabolism in the left thalamus (white arrow). Figure adapted from Hellem et al. (Hellem, 2021) under the Creative Commons Attribution License.
Dopaminergic Imaging Markers
The degeneration of MSNs in the striatum is a defining feature of HD, leading to dysfunction in dopamine receptor activity. PET imaging using tracers targeting dopamine D1 and D2/3 receptors provides insights into the progression of the disease. For example, studies using the [11C]SCH-23390 tracer show a significant reduction in D1 receptor density in the putamen of individuals with manifest HD, with a decrease of about 50% compared to healthy controls (Cybulska, 2020). Longitudinal studies have demonstrated that D1 receptor loss occurs more gradually in premanifest HD, with a slower annual decline rate of 2%, compared to a faster rate of 5% per year in individuals with manifest HD (Cybulska, 2020).
Similarly, PET imaging with [11C]raclopride, a PET tracer for the quantification of D2/3 receptors, has shown significant D2 receptor loss in the striatum, which varies by disease stage, with premanifest HD showing a 10-50% loss, compared to 40-60% in manifest HD (Fazio, 2018). Moreover, in manifest HD, reduced D2/3 binding in the cortex correlates with poorer performance on neuropsychological tests, particularly those assessing verbal fluency and memory (Cybulska, 2020).
PET imaging studies using presynaptic markers, such as [11C]dihydrotetrabenazine (DTBZ) reveals reduced vesicular monoamine transporter 2 (VMAT2) binding in the striatum of manifest HD patients. Additionally, [11C]β-CIT PET scans have demonstrated a 50% reduction in dopamine transporter (DAT) binding in the striatum, further supporting the role of dopaminergic dysfunction in HD pathology (Fazio, 2018).
Phosphodiesterase 10A (PDE10A) Imaging
PDE10A is an enzyme highly expressed in MSNs that can be monitored using PET imaging with ligands such as [18F]JNJ42249152, [18F]MNI-659, and [11C]IMA-107 (Fazio, 2018). In manifest HD, PET imaging reveals a significant reduction in striatal binding, which correlates with disease severity (Cybulska, 2020). Longitudinal studies have shown that this reduction is more pronounced in the putamen, caudate nucleus, and globus pallidus compared to healthy controls, with greater annual rates of decline (Cybulska, 2020).
Interestingly, in premanifest HD, increased binding has been observed in the motor thalamic nuclei, suggesting a compensatory response that diminishes as symptoms manifest (Cybulska, 2020). These early PDE10A alterations can be detected up to 25 years before the onset of clinical symptoms and may serve as a predictive biomarker for disease onset, offering a potential target for intervention (Niccolini, 2015).
Neuroinflammation Markers
Neuroinflammation is another hallmark of HD, with microglial activation serving as a key indicator. PET imaging using tracers that target the 18-kDa translocator protein (TSPO), such as [11C]PK11195, has shown increased radioligand binding in both premanifest and manifest HD, suggesting early-stage microglial activation as a potential biomarker for the disease (Cybulska, 2020). Increased binding has been observed in several brain areas, including the striatum, cortex, globus pallidus, and insula, regions involved in motor, cognitive, and psychiatric functions (Cybulska, 2020). This neuroinflammatory marker could prove useful for patient stratification in clinical trials aimed at disease-modifying therapies, potentially aiding in early identification of individuals at risk of symptomatic conversion (Hobbs, 2024).
Overall, HD is a complex neurodegenerative disorder, and advances in neuroimaging have significantly enhanced our understanding of its early progression. PET imaging has revealed key biomarkers related to glucose metabolism, dopaminergic activity, phosphodiesterase expression, and neuroinflammation. These insights not only help track disease progression, but also offer potential targets for therapeutic interventions.
How do PET imaging biomarkers correlate with traditional clinical outcome measures in HD?
PET imaging provides a powerful tool for tracking early disease progression in HD, closely correlating with clinical outcome measures. For instance, FDG PET imaging in premanifest HD shows a strong negative correlation between striatal metabolism and the CAG and age product (CAP) score. This observation suggests that as individuals approach motor symptom onset, metabolic activity in the striatum decreases (Hellem, 2021). Interestingly, metabolism in the striatum was found to explain more of the variation in CAP scores than MRI volume measurements, highlighting the sensitivity of PET in tracking disease progression at earlier stages (Hellem, 2021).
In addition to striatal changes, progressive cortical hypometabolism in premanifest HD has been shown to correlate with the progression of cognitive deficits (Fazio, 2018). Hypometabolism in the caudate and putamen of premanifest HD carriers is linked to a higher risk of symptomatic conversion (Hobbs, 2024). Cross-sectional data also suggests a correlation between basal ganglia hypometabolism and slower psychomotor speed in premanifest carriers (Hobbs, 2024). In manifest HD, both striatal and cortical hypometabolism correlate with functional capacity, motor function, verbal learning, memory, and overall cognitive impairment (Hobbs, 2024). Additionally, cortical hypometabolism in both premanifest and manifest stages is associated with difficulties in arithmetic word-problem solving, while changes in cortical and limbic metabolic networks in early manifest HD correlate with apathy.
In JHD, greater annual changes in glucose metabolism have been linked to worsening dystonia, parkinsonism, and reduced independence (Caligiuri, 2024). PET imaging studies have also shown that decreased striatal D2 receptor binding in premanifest HD correlates with executive dysfunction and poorer verbal fluency, while PDE10A binding loss in both premanifest and manifest stages is associated with motor impairment (Hobbs, 2024).
What are the future directions for PET imaging in HD clinical trials?
One of the most promising future directions in HD research is the development of PET radioligands that bind specifically to mHTT aggregates. These radioligands could play a critical role in clinical trials aimed at reducing mHTT levels, allowing researchers to visualize and quantify cerebral target engagement and thereby providing insights into regional distribution (Lieberman, 2022; Hobbs, 2024). However, the challenge lies in the relatively low abundance of mHTT aggregates compared to misfolded proteins in other neurodegenerative diseases, such as Alzheimer's disease (AD) and Parkinson’s disease (PD) (Dickmann, 2024). To overcome this issue, high-affinity ligands that are highly selective for mHTT over other protein aggregates like TDP-43, alpha-synuclein, tau, and amyloid-beta, must be developed (Dickmann, 2024).
Several promising PET ligands have been tested for their ability to target mHTT, including [11C]CHDI-180R, [11C]CHDI-626, [18F]1, and [18F]CHDI-650. Despite their potential, many of these ligands have shown limited selectivity and affinity for mHTT, with some being discontinued due to insufficient efficacy (Dickmann, 2024). To address this issue, continued research is focused on designing and evaluating novel PET radioligands capable of reliably targeting mHTT in vivo (NCT06634628). Successful development of these radioligands would provide a non-invasive method for detecting and monitoring of mHTT aggregates, a critical advancement for both clinical trials and early diagnosis of HD (Kaur, 2021).
Moreover, these radioligands could offer invaluable insights into disease progression, enabling more precise patient stratification in clinical trials. They may also play a pivotal role in evaluating responses to disease-modifying treatments targeting mHTT, improving treatment efficacy (Kaur, 2021).
In HD, the accumulation of mHTT protein aggregates, particularly in the striatum, leads to neurodegeneration and is associated with motor, cognitive, and neuropsychiatric impairments. PET radioligands with high affinity and selectivity for mHTT are still under development, but hold promise for objective quantification of mHTT burden in the brain. These tools could provide a crucial marker for tracking disease progression and evaluating novel therapies targeting mHTT in clinical trials, thereby advancing both diagnostic and therapeutic strategies.
Click to copy link
Our team would be happy to answer any questions about PET Biomarkers in Clinical Trials of Huntington’s Disease or provide specific information about our other Imaging services.
Discover more about our Imaging Services
Related Content
Up-to-date information on best practices related to the use of neuroimaging in clinical trials of neurological diseases.
[18F]DOPA PET in Parkinson’s Disease Clinical Trials
How [18F]DOPA PET is used to monitor disease progression & response to therapeutic intervention in Parkinson’s disease & movement disorders clinical trials.
Spinocerebellar Ataxia Clinical Trials & Imaging Biomarkers
An overview of structural MRI, DTI and MRS imaging biomarkers to monitor disease progression in spinocerebellar ataxia clinical trials.
MRI Biomarkers in Clinical Trials of Huntington’s Disease
An overview of the use of structural and diffusion MRI imaging biomarkers for Huntington’s disease (HD) clinical trials.
Neuroimaging Biomarkers for Tuberous Sclerosis Complex (TSC)
Overview of brain structural MRI and diffusion-weighted imaging biomarkers to monitor efficacy of disease-modifying therapeutics in clinical trials of TSC.
Modeling Protein Misfolding in Neurological Diseases
Computational modeling of protein misfolding mechanisms in neurodegeneration and the application to discovery & development of disease-modifying therapeutics.
Imaging Biomarkers for Friedreich’s Ataxia Clinical Trials
An overview of the use of MRI and DTI imaging biomarkers for Friedreich’s ataxia (FRDA) in research studies & multi-center clinical trials.
MRI in Clinical Trials of Multiple System Atrophy (MSA)
This resource provides an overview of the utility of volumetric MRI and diffusion-weighted imaging (DWI) as biomarkers in research studies of MSA.